Prof. Juan Yang's team recently published collaborative work in direct ammonia fuel cells with the Universities of SUNY-Buffalo and Virginia Tech from the USA and Nanjing University.
Green ammonia, a carbon-free energy carrier, holds representative superiorities relative to hydrogen, such as being easier to be liquified, transported and stored, difficult to ignite, and safer for practical applications. If green ammonia is directly supplied at the anode in a fuel cell to generate electricity, the products are only water and nitrogen with no carbon emissions. The hydrogen economy is stepping forward to the ammonia economy with the deep evolution of green energy.
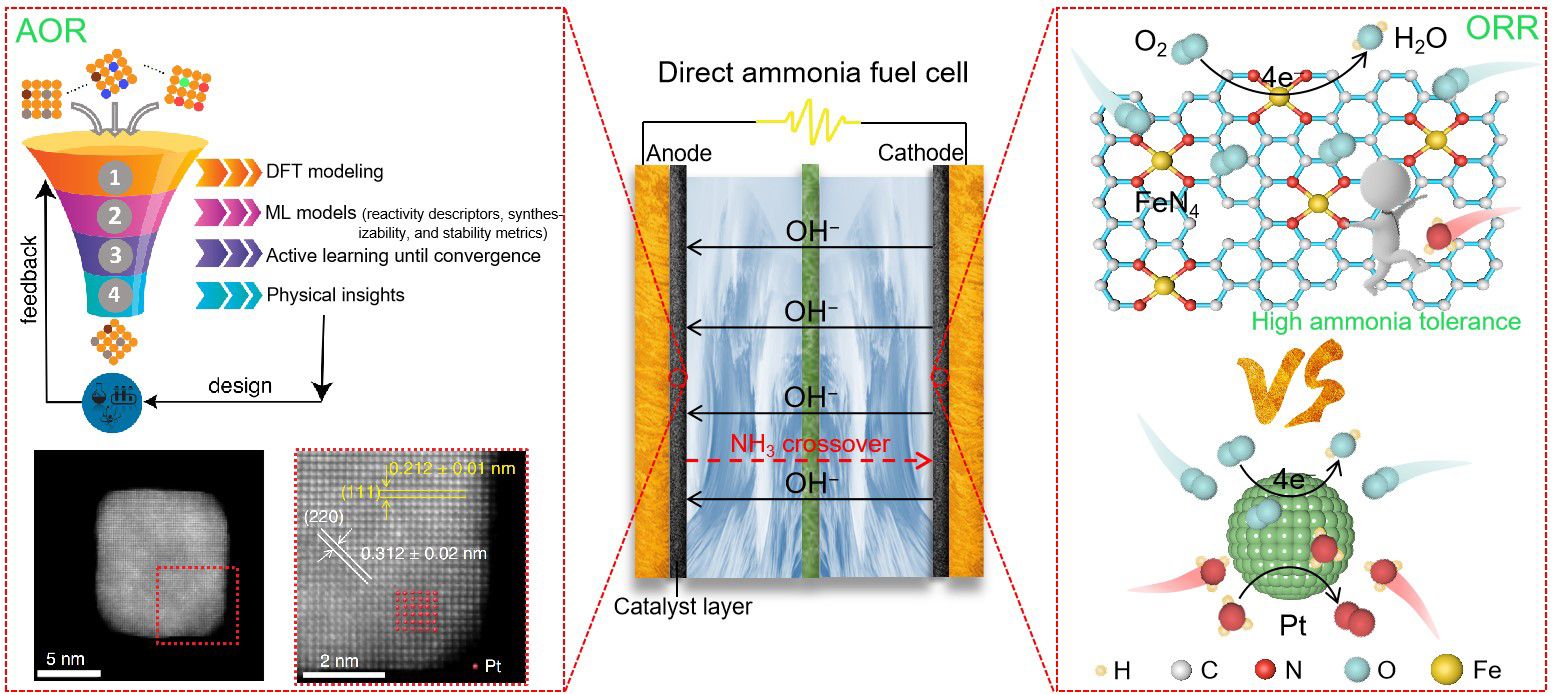
Prof. Juan Yang and Dr. Yi Li from Jiangsu University, Prof. Hongliang Xin from Virginia Tech, and Prof. Gang Wu from SUNY-Buffalo have collaborative research achievements in the design and synthesis of novel ammonia oxidation electrocatalysts for direct ammonia fuel cell anodes. This collaborative work, entitled "Interpretable design of Ir-free trimetallic electrocatalysts for ammonia oxidation with graph neural networks", has been published in Nature Communications, a reputable Nature-based journal.
Prof. Xin's team contributed to a new modelling method of graph neural networks for promising catalyst prediction, while Prof. Yang, Dr. Li and Prof. Wu contributed to the experimental synthesis of the predicted Ir-free trimetallic alloys such as Pt3Ru-M (M = Fe, Co or Ni). More significantly, model interpretations enabled by theory integration in deep learning uncover a crucial physical factor, for instance, adsorbate resonance energies of frontier orbitals, in governing the reactivity of d-metal alloy surfaces and shed light on strategies for designing high-performance catalytic systems beyond the d-band centre metric of binding sites.
An oxygen reduction reaction occurs at the cathode of a direct ammonia fuel cell, which is a four-electron reaction process. Although the kinetics of a cathodic reaction is faster than that of the anode, it suffers from interference by ammonia, which can inevitably be permeated from the anode to the cathode through the anion exchange membrane under working conditions. In addition, the oxygen reduction and ammonia oxidation reactions may simultaneously occur on the catalyst surface due to the same operating potential window, leading to the parasitic current generation. This current would reduce fuel utilization and degrade the overall performance of direct ammonia fuel cells and devices.
Dr. Zhongti Sun and Dr. Li from Prof. Yang's team and Prof. Bing Wang from Nanjing University have collaborative work on atomically dispersed M-N-C (M = Fe, Co or Mn) oxygen reduction catalysts. Experimental and theoretical results have proved that this class of M-N-C catalyst can tolerate ammonia interference under oxygen-reduction conditions, whereas the classic platinum carbon catalyst adsorbs ammonia, leading to irreversible activity loss. This collaborative work entitled "Ammonia tolerance of atomically dispersed single metal site catalysts: Mechanistic understanding and high-performance oxygen reduction electrocatalysis" has been published in Advanced Functional Materials, a reputable journal in material science. The first author is Zirui Wu, a Ph.D. candidate from Prof. Yang's team.
The above new findings may pave the way for the development of direct ammonia fuel cells. These works have been supported by the National Natural Science Foundation of China, the Natural Science Foundation of Jiangsu Province, and the China Postdoctoral Science Foundation.
Publication links:
https://www.nature.com/articles/s41467-023-36322-5
https://onlinelibrary.wiley.com/doi/10.1002/adfm.202301084